Solar flares, powerful bursts of radiation originating from the Sun, have long intrigued scientists due to their potential impacts on space weather and Earth’s technological systems. This article delves into the evolution of solar flare research, tracing its roots from early observations to the sophisticated techniques employed today. By examining key findings from recent investigations, we gain insight into the mechanisms driving these energetic events and their effects on our planet. Despite significant advancements, the study of solar flares remains fraught with challenges, prompting ongoing exploration and innovation. Looking ahead, future research aims to enhance our understanding and predictive capabilities, ensuring better preparedness for solar-induced disruptions.
uzocn.com offers a detailed exploration of this topic.
1. Introduction to Solar Flares
Solar flares are powerful bursts of radiation originating from the Sun’s atmosphere, specifically from areas with intricate magnetic fields near sunspots. These events occur when magnetic energy accumulated in the solar atmosphere is abruptly released, generating a broad range of electromagnetic radiation. Solar flares are classified by their intensity, with the weakest being C-class flares and the most powerful being M- and X-class flares, which can have substantial impacts on space weather.
Solar flares, immense bursts of energy from the Sun, can accelerate particles to nearly the speed of light. This triggers a chain reaction with far-reaching consequences across the solar system. On Earth, these flares can disrupt essential technologies like radio communications, GPS signals, and even power grids, threatening both ground-based and spaceborne systems. Therefore, understanding the mechanisms behind solar flares is vital to predicting these events and minimizing their potential impact on our technologically advanced society.
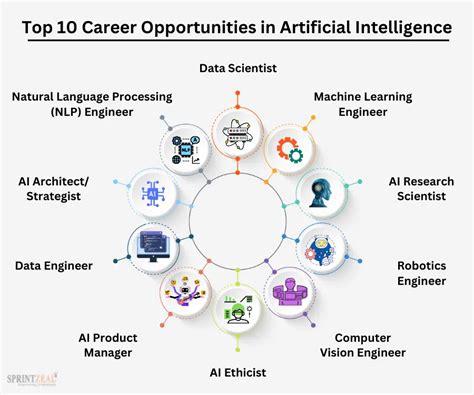
2. Historical Investigations
The study of solar flares has roots in the 19th century, when astronomers began observing their striking effects. The earliest documented solar flare, the Carrington Event of 1859, was named after British astronomer Richard Carrington, who witnessed a colossal solar flare followed by a geomagnetic storm. This event brought to light the potential influence of solar activity on Earth’s magnetic environment, laying the groundwork for subsequent research in the field.
Early 20th-century technological leaps, including the development of photographic plates and spectrographs, enabled scientists to observe and analyze solar flares with unprecedented detail. The advent of radio telescopes in the 1940s further transformed solar flare research, uncovering the intricate radio emissions that accompany these powerful eruptions.
The 1960s and 1970s witnessed a transformative leap in solar research with the advent of space-based observatories. The launch of missions like the Solar and Heliospheric Observatory (SOHO) provided unparalleled views of the Sun, unhindered by Earth’s atmosphere. These missions revolutionized our understanding of solar flare dynamics and their connection to coronal mass ejections (CMEs). By establishing the fundamental characteristics and impacts of solar flares, historical investigations paved the way for contemporary research. This legacy continues to inspire the development of more sophisticated studies and technologies to further explore the Sun’s powerful phenomena.
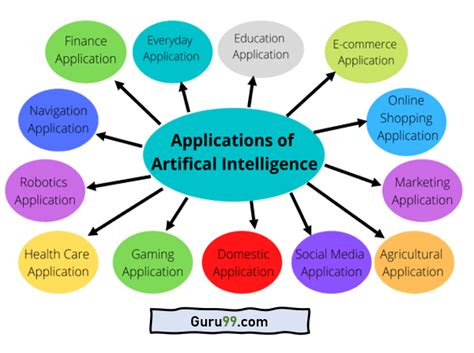
3. Modern Techniques in Solar Flare Research
Modern solar flare research employs a range of advanced techniques that provide deeper insights into these powerful events. Space-based observatories, such as the Solar Dynamics Observatory (SDO) and the Solar and Heliospheric Observatory (SOHO), use high-resolution imaging and spectroscopy to capture detailed observations of solar flares and their associated phenomena. These satellites offer continuous, real-time monitoring of the Sun, revealing intricate magnetic field interactions and flare dynamics.
Ground-based radio telescopes contribute by detecting and analyzing the high-energy radio waves emitted during solar flares. These observations help identify the acceleration mechanisms of solar particles. Additionally, advanced computational models and simulations are employed to interpret data and predict flare activity. Researchers use machine learning algorithms to analyze vast datasets, identifying patterns and improving predictive capabilities. Together, these modern techniques enhance our understanding of solar flares, their origins, and their potential impacts on space weather
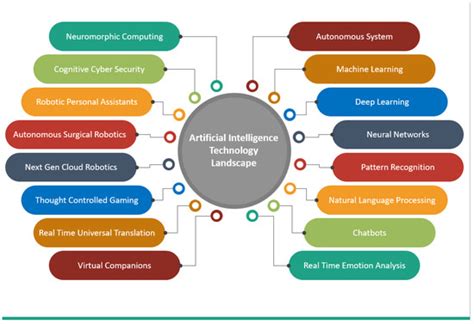
4. Key Findings from Recent Studies
Recent studies have significantly advanced our understanding of solar flares and their effects. One key finding is the intricate relationship between solar flares and coronal mass ejections (CMEs). Research has shown that intense solar flares often precede or accompany CMEs, with their interactions influencing the intensity of geomagnetic storms on Earth.
Additionally, advancements in imaging technology have revealed detailed structures within solar flares, such as loop-like structures and magnetic reconnection sites, which play crucial roles in flare dynamics. Recent data also highlights the role of solar flares in accelerating high-energy particles, contributing to space weather phenomena and posing risks to satellites and astronauts.
Studies have also improved our knowledge of the Sun’s magnetic field configuration, revealing how solar magnetic fields can store and release energy during flares. These findings have enhanced our ability to predict flare activity and mitigate its impacts on technology. Overall, recent research provides a more comprehensive picture of solar flare mechanisms and their broader implications for space weather and Earth’s technological systems.
5. Impacts of Solar Flares on Earth
Solar flares have a substantial impact on Earth, primarily through their influence on space weather. A solar flare releases a surge of radiation that can disrupt radio communications and GPS signals. This interference can impact various systems, including satellite navigation and emergency communication networks. Furthermore, high-energy particles accelerated by solar flares pose risks to astronauts and spacecraft. These particles can damage sensitive electronics and jeopardize mission safety.
Furthermore, solar flares can interact with Earth’s magnetic field, causing geomagnetic storms. These storms can induce electrical currents in power grids, potentially causing power outages and infrastructure damage. The enhanced ionization in the upper atmosphere during a flare can also intensify auroras, resulting in more vibrant and widespread displays of the northern and southern lights.
The widespread consequences of these disruptions highlight the critical need for ongoing research. This research aims to predict and minimize the effects of solar flares, thereby safeguarding the stability of our technology-reliant society.
6. Challenges in Solar Flare Research
Solar flare research faces several challenges that complicate our understanding of these powerful events. One major challenge is the unpredictable nature of solar flares. Despite advances in monitoring technology, accurately forecasting when and where flares will occur remains difficult due to the complex and dynamic nature of solar magnetic fields.
Another challenge is the vast amount of data generated by modern instruments. Analyzing this data to discern meaningful patterns and connections requires significant computational resources and sophisticated algorithms. Ensuring that these tools and models can accurately interpret the data is an ongoing challenge.
Additionally, the impacts of solar flares on Earth are influenced by a variety of factors, including the orientation of the Sun’s magnetic field and the Earth’s magnetic environment. This variability complicates efforts to predict the exact effects of solar flares on technology and infrastructure. Addressing these challenges requires continued advancements in observational technology, data analysis techniques, and predictive modeling.
7. Future Directions in Solar Flare Research
The future of solar flare research promises exciting advancements and new directions. One key area of focus is enhancing predictive capabilities through improved observational technologies. Upcoming missions, such as the Parker Solar Probe and the European Space Agency’s Solar Orbiter, are designed to provide unprecedented close-up views of the Sun, offering detailed insights into solar magnetic fields and flare dynamics. These missions aim to refine our understanding of the mechanisms behind solar flares and their relationship with coronal mass ejections.
Additionally, researchers are exploring the integration of machine learning and artificial intelligence to analyze vast datasets more efficiently. These technologies can identify patterns and anomalies that might be missed by traditional methods, improving flare prediction models and risk assessments.
Another promising direction involves better understanding the interaction between solar flares and Earth’s magnetosphere. Advances in space weather forecasting could enhance our ability to predict and mitigate the impacts of solar flares on technological systems and infrastructure.
Finally, interdisciplinary collaboration is crucial, as insights from physics, engineering, and space science come together to address the complex challenges of solar flare research. Continued innovation in both observational techniques and data analysis will be key to advancing our knowledge and preparedness for solar activity.
Solar flares remain a fascinating and complex area of study with significant implications for space weather and technology. Historical investigations have laid a strong foundation, while modern techniques continue to enhance our understanding. Despite challenges, ongoing research and future advancements promise to improve our predictive capabilities and mitigation strategies. By addressing these challenges and exploring new directions, we can better prepare for and respond to the impacts of solar flares on Earth.
uzocn.com